RESEARCH INTERESTS
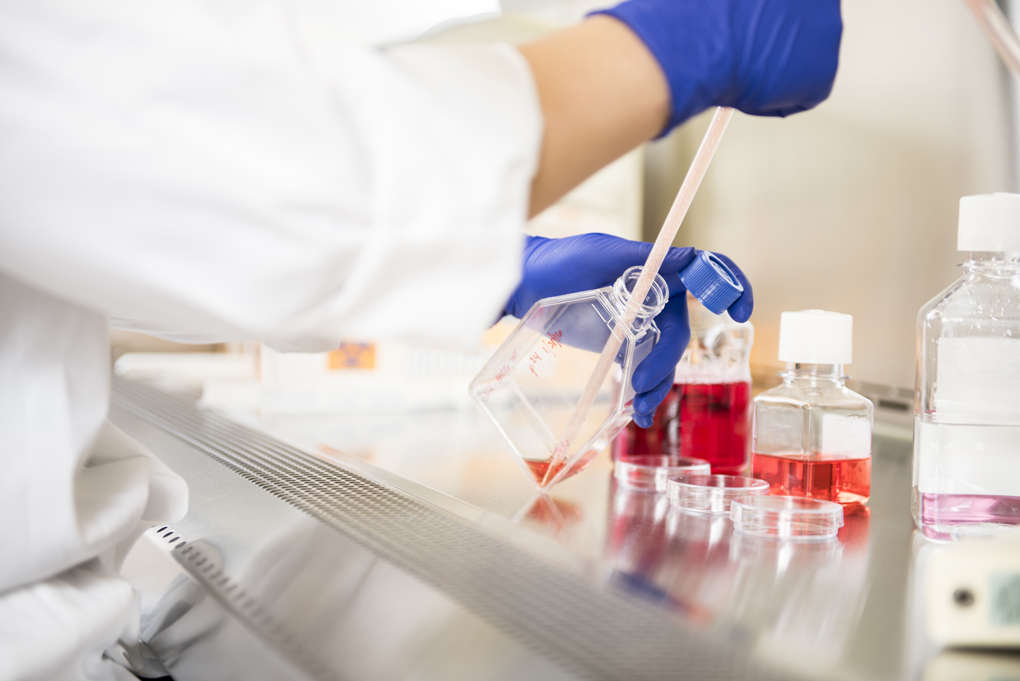
It is now widely understood, at least among gerontologists, that when we speak of aging we speak of the time-dependent disruption of a loosely-defined collection of cellular and physiological processes resulting in impairment of an organism's ability to maintain homeostasis. At the cellular level, key processes that become dysfunctional with time, and which are now known to contribute to aging, include genomic maintenance, telomere length regulation, epigenetic regulation, proteostasis, nutrient sensing, mitochondrial electron transport chain function, senescent cell production, stem cell maintenance and intercellular communication. As time progresses, the cumulative disruption of these "hallmark" processes of aging becomes greater and greater such that individuals become increasingly sensitive to homeostatic perturbation. This so-called "homeostenosis of aging", or the narrowing over time of the limits to which an organism can be pushed before it reaches a point from which it cannot recover and survive, is in fact the greatest risk factor for many of the diseases we commonly associate with aging. Simply said - "growing old" moves us into metabolic and physiologic states that increasingly elevate our risk for getting age-related diseases. Among these diseases are cardiovascular disease, most cancers, kidney disease, diabetes and dementias. These, as you will perhaps recognize, are today's major causes of mortality for people living in the western world. Interventions that prevent aging, or more correctly homeostenosis, therefore hold the greatest potential for reducing the burden of disease among the elderly because they are likely to simultaneously delay or prevent most age-related conditions. Targeting some of the hallmark processes of aging is a major focus of the Rea Lab.
Broadly speaking, we can distinguish two forms of mitochondrial dysfunction in patients - frank dysfunction, as seen in the often fatal congenital mitochondrial syndromes (estimated to affect ~1/4500 live births), and the more insidious decline that is associated with aging. Indeed, studies have shown that with age, the Km and Vmax of complexes I, III and IV of the mitochondrial ETC all decline with time. As well, the abundance of ubiquinone and cytochrome c, two mobile electron carriers within the chain, both become less; and ATP production decreases at the expense of elevated reactive radical species production. Whether causative or consequential, age-associated mitochondrial dysfunction is linked with many diseases that limit life-expectancy in humans, including Type II diabetes, metabolic syndrome, Alzheimer's disease, Parkinson's disease, depression and blindness. The pronounced role of mitochondria in the pathogenesis of multiple diseases is a consequence of their role in an extensive list of essential cellular processes. The mitochondrial electron transport chain (ETC) alone generates up to 90% of cellular ATP. Other important functions of mitochondria include calcium sequestration, Fe-S cluster formation, nucleotide biosynthesis, infection signaling, stem cell maturation, apoptosis signaling, oxidative stress signaling, steroid biosynthesis and xenobiotic detoxification. Clearly, mitochondria are essential organelles and their proper function is required for both health and longevity. The nematode Caenorhabditis elegans has proven to be a powerful non-mammalian model system for studying the basic mechanisms of aging. Many of the fundamental discoveries regarding genetic pathways that regulate aging have come from research using C. elegans, including key insights into the roles of insulin-like signaling, sirtuins, mTOR signaling, hypoxia, proteostasis, autophagy, and epigenetic modifiers of aging. C. elegans has been a particularly powerful model for understanding the role of mitochondrial dysfunction in aging and in this regard our lab has been a major driving force in this research area.
While complete loss-of-function of the mitochondrial ETC is lethal in most species, including worms and mice alike, numerous studies have underscored the surprising finding that partial ETC inhibition often leads to life extension. Indeed, worms seem to be particularly good at extending their lifespan when their mitochondrial ETC is partially perturbed. It is not uncommon, for example, to observe a 30% increase in adult survival time, for some perturbations it can be as great as three fold greater than wild type animals. Two decades of research have revealed that the long-lived mitochondrial disruptants of C. elegans employ a variety of "retrograde responses" to extend their life. Retrograde responses are signals that originate from compromised mitochondria but act within the nucleus to orchestrate adaptive gene-expression changes to resolve or reduce mitochondrial stress. In some cases these retrograde responses also act hormetically and continue to work to extend life. Higher eucaryotes have evolved different kinds of retrograde responses that are triggered by a variety of mitochondrial stressors, including depletion or mutation of mtDNA, reduced ETC activity, reduced mtDNA translation, increased oxidative stress, misfolded protein aggregation, changes in mitochondrial turnover and even exposure to bacterial toxins. Depending on the particular ETC lesion, specific Mit mutants may activate one or many retrograde responses. The Mit class of ETC disruptants are therefore really a heterogeneous group of longevity mutants sharing only the commonality of adopting different metabolic strategies that bypass their compromised mitochondrial function and which fortuitously all optimize the status quo between key mitochondrial parameters and long life.
We recently discovered a novel mitochondrial retrograde response in C. elegans that extends life and is unrelated to any known longevity pathway previously described. We mapped the key signaling elements of this pathway to a previously uncharacterized MAPK cascade comprised of DLK-1, SEK-3 and PMK-3. We also showed that the atypical β-tubulin, tbb-6, is a potent marker of pathway activation. Our newest findings reveal that this novel retrograde response has multiple signaling branches that control discrete cellular and organismal phenotypes, including lifespan. Currently the Rea lab is focused on understanding the mechanism by which this novel retrograde response is triggered, how it functions to extend life, if it can be manipulated in the absence of ETC disruption to extend life, and whether the mechanisms we discover can be translated to humans.
Cellular senescence is a form of cell cycle arrest that arises in response to different types of stimuli. It can be viewed either favorably or unfavorably, depending on the context in which it occurs. During both embryonic development and wound repair, senescent cells play an important role in the tissue remodeling process. Cellular senescence also functions as a cancer preventative measure following DNA damage. These positive roles are counterbalanced by the negative effects caused by the excessive and aberrant accumulation of senescent cells in aged tissue. In this setting, senescent cells impair tissue regeneration and, as well, create a pro-inflammatory milieu favorable to the onset and progression of various age-related diseases, including cancer. Genetic engineering studies within the last five years have shown that removal of senescent cells in aged mice is highly beneficial. Specifically, whole-body ablation of cells expressing p16(Ink4a), a gene often associated with cellular senescence, results in enhanced renal and cardiac function and extends life. Drug studies targeting senescent cells reveal similar findings. These exciting results suggest therapeutic approaches targeting senescent cells in humans could be a powerful means to allay the negative consequences of aging among the US population and indeed several companies have already begun moving into this space. In the Rea lab we are working on novel approaches to therapeutically target senescent cells in mammals. We are making use of cutting edge technology to define the types of senescent cells that occur in vivo, and the conditions leading to different senescence programs across tissues. We are also determining how different senescence programs are related to the risk of oncogenesis. Finally, we are designing novel strategies for the clearance of senescent cells.
Our lab makes full use of the powerful genetic and scientific tools that are available for C. elegans research. Use of C. elegans affords many benefits, including a short lifespan, ease of manipulation, and low experimental cost. These attributes are reflected in the range of experiments that we are currently undertaking - from construction of multiple fluorescent reporter strains using CRISPR/Cas9 and other genetic engineering techniques to tissue-specific phosphoproteomic analyses. Recently we teamed up with the UW spin-off company GeroTech to serve as a beta test site for commercialization of a high-throughput, automated robotic system to assess lifespan in C. elegans and related species. This breakthrough piece of technology affords us an opportunity to perform the first truly large-scale, unbiased genetic and pharmacological screens for increased lifespan in worms. The 1000 fold increase in processivity of worm lifespan analysis will speed up the pipeline for moving drug candidates from the bench to the bedside.
Aging is a complex process that affects almost every species, albeit at different rates. Oddly, even within species, individuals age at markedly different rates. Genetically identical individuals cultured under environmentally identical conditions, for example, exhibit large differences in life span. Understanding the basis for such life span variance remains an ongoing effort in the Rea Lab. We have been using systems biology tools from the chemical engineering field to help us understand mechanisms of cellular aging. As a test-bed, we have focused on the single-celled yeast Saccharomyces cerevisiae. We have exploited in silico models of intermediary metabolism that contain several thousand reactions and asked if metabolic configuration per se can alter lifespan. Our most recent studies have identified metabolic states associated with extended survival in this species.